Author: Deniz Usta
The kidneys, essential paired organs within the mammalian body, are situated symmetrically on either side of the dorsal abdomen, with the right kidney positioned on the right flank and the left kidney on the left flank.
These remarkable organs play a pivotal role in maintaining the body’s internal environment by performing the crucial function of waste product filtration from the bloodstream. This intricate process of filtration transpires as blood courses through a specialized structure known as the glomerulus. Remarkably, the specific anatomical configuration of kidneys can exhibit noteworthy variations across different species, reflecting the remarkable adaptability and diversity of life forms in the animal kingdom.
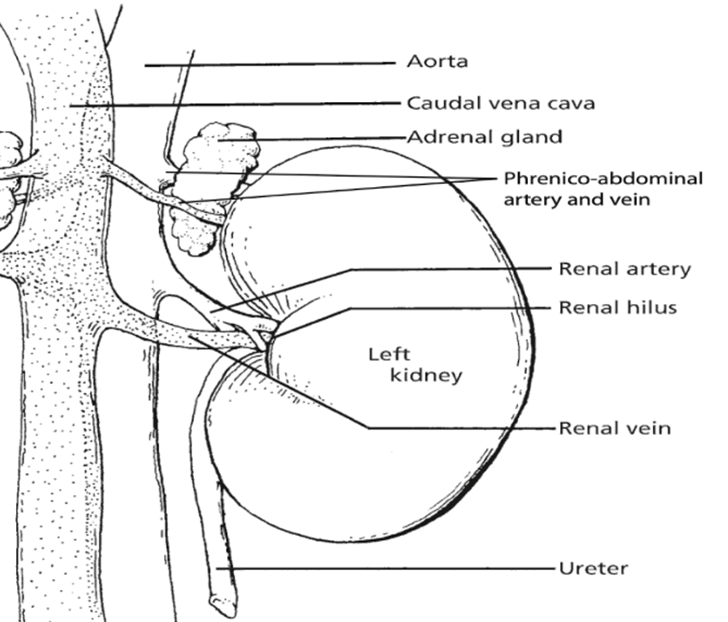
The Nephron
The nephron, a structurally and functionally intricate unit, serves as the fundamental building block of the kidney’s physiological operations. One intriguing aspect of nephron biology is the considerable interspecies variation in their abundance. Nephrons can be broadly classified into two main categories: cortical or corticomedullary nephrons and juxtamedullary nephrons. The former, found predominantly in the outer and middle cortical regions, are characterized by the presence of glomeruli, and are associated with Henle loops that extend to the junction between the medulla and cortex, or even into the outer zone of the medulla. In contrast, juxtamedullary nephrons, as the name suggests, are situated nearer to the medulla within the renal cortex. These nephrons are distinguished by glomeruli as well and are connected to loops of Henle that extend deep into the medulla, with some Henle loops reaching as far as the renal pelvis. This structural diversity in nephron distribution and configuration underlines the remarkable adaptability of the renal system across species, allowing it to finely tune its physiological functions to meet the unique demands of each organism.
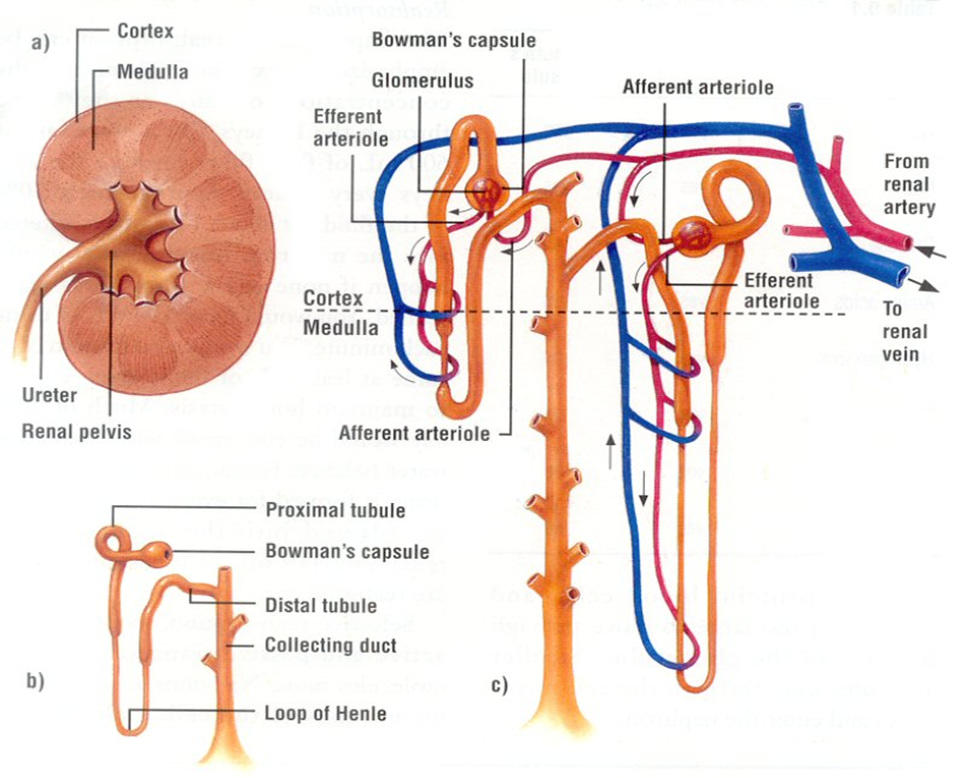
The kidneys, apart from their role in waste filtration, perform a multifaceted function that extends to the secretion of hormones critical for regulating blood pressure, red blood cell production, and mineral metabolism. These hormones, namely the renin-angiotensin system (RAS), erythropoietin (EPO), and 1,25-dihydroxy-vitamin D3, are pivotal for maintaining various aspects of bodily homeostasis.
The renin-angiotensin-aldosterone system (RAAS) orchestrates a complex interplay of physiological processes. It actively governs the body’s electrolyte balance, fluid equilibrium, and blood pressure regulation, thereby playing a vital role in cardiovascular health.
Erythropoietin (EPO), another significant hormone produced by the kidneys, serves as a key player in the body’s response to low oxygen levels. In situations such as high altitudes or in individuals with chronic lung disorders, EPO stimulates the bone marrow to boost the production of red blood cells. The kidneys respond to hypoxia by releasing more EPO, enhancing the oxygen-carrying capacity of the blood. In medical practice, EPO is often prescribed as a therapeutic agent to address anaemia.
The maintenance of calcium and phosphorus levels in the body is heavily influenced by 1,25-dihydroxy-vitamin D3. This hormone exerts its effects on multiple fronts, influencing the intestines to facilitate the absorption of calcium and phosphorus from dietary sources, regulating calcium deposition and resorption in bones, and controlling the production of parathyroid hormone (PTH). PTH is integral in preserving normal blood calcium levels, further emphasizing the kidneys’ role in mineral metabolism.
To execute these vital functions, kidney cells, like all cells in the body, require energy. The kidneys, in fact, stand as one of the most energy-demanding organs, surpassed only by the heart. This high energy requirement is attributed to their substantial mitochondrial content and oxygen consumption. Kidneys maintain a high resting metabolic rate, necessitating a profusion of mitochondria to meet their energy needs.
Glucose, drawn from the bloodstream, serves as a primary energy source for kidney cells. In addition to glucose, kidney cells can also utilize alternative energy substrates, including fatty acids and amino acids. This metabolic versatility is essential for the kidneys to adapt to varying energy demands and maintain their multifaceted physiological roles.
Glucose: The Key Fuel for ATP Synthesis
Aerobic respiration is a fundamental cellular process wherein oxygen is meticulously consumed to produce adenosine triphosphate (ATP), water, and carbon dioxide (CO2). The lion’s share of ATP generated during aerobic respiration is a direct consequence of the intricate workings of the electron transport chain (ETC) and the sequential movement of electrons within this system. The process commences with glycolysis, the initial step of aerobic respiration, which involves the conversion of glucose, the key fuel, into pyruvate.
Within the mitochondrial matrix, the pyruvate dehydrogenase complex enzymatically converts pyruvate to acetyl-CoA, a crucial substrate that fuels the tricarboxylic acid (TCA) cycle. This cycle results in the production of six molecules of nicotinamide adenine dinucleotide (NADH+H+), two molecules of flavin adenine dinucleotide (FADH2), and six molecules of carbon dioxide and water for each glucose molecule processed.
In the inner mitochondrial membrane, complex I and complex II of the ETC come into play, receiving electrons derived from NADH+H+ and FADH2, respectively. These electrons embark on an intricate journey through the electron transport chain, moving from one complex to another, until they reach complex IV. At complex IV, oxygen serves as the final electron acceptor, thus facilitating the formation of water as the terminal product.
Notably, coenzyme Q and cytochrome c, essential components situated within the inner mitochondrial membrane, play a pivotal role in mediating the transfer of electrons from complex I/II to complex III and complex III to complex IV, respectively, ensuring the seamless progression of electron flow.
Ultimately, the conversion of adenosine diphosphate (ADP) to ATP is catalyzed by ATP synthase, a dynamic enzyme complex. This enzyme harnesses the energy generated by the electron transport chain to drive the synthesis of ATP, making it a central player in the overall process of aerobic respiration, as illustrated in Fig. 3.
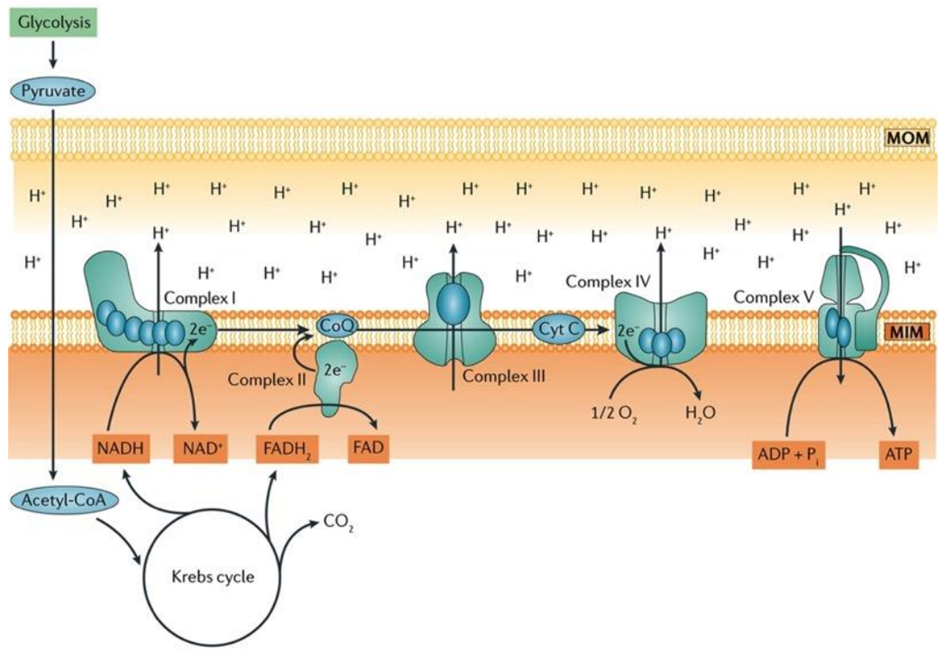
Fatty Acids: Powering the Kidney as Efficient Fuels
Proximal tubule cells rely on aerobic respiration, the most efficient mechanism for ATP production, as their energy demands are substantial due to the necessity of powering ion transport processes. Notably, fatty acids emerge as a significant energy source for proximal tubules, as a single molecule of palmitate yields a higher ATP output compared to a molecule of glucose.
Proximal tubule cells can perform one of two key functions related to fatty acids: either they synthesize fatty acids in their cytoplasm, activating them with coenzyme A before transferring them into mitochondria through the carnitine shuttle, or they can uptake fatty acids using specialized transport proteins, like platelet glycoprotein 4, also known as CD36.
In the kidney’s proximal tubule cells, the transformation of fatty acids into ATP is a complex and vital process. After the uptake or synthesis of fatty acids, these molecules need to be metabolized to yield energy. Fatty acids are first transported into the mitochondria via the carnitine shuttle, where they undergo a series of enzymatic reactions. These reactions break down the fatty acids through beta-oxidation, a process that progressively shortens the carbon chains of the fatty acids. As a result, acetyl-CoA molecules are generated from the breakdown of fatty acids. Acetyl-CoA then enters the citric acid cycle (also known as the Krebs cycle), a central metabolic pathway in mitochondria. In the citric acid cycle, acetyl-CoA is further processed to produce high-energy electrons and other intermediates. These high-energy electrons are transferred to the ETC, where they drive a series of redox reactions that ultimately lead to the production of ATP. This ATP can then be used by the kidney cells to carry out various essential functions, including the reabsorption of nutrients and the regulation of fluid and electrolyte balance within the body.
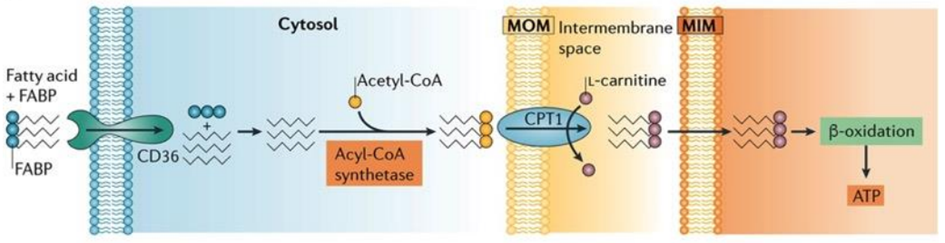
The Role of Amino Acids as Fuels for Kidney Cells
Amino acid transporters are abundantly present in the luminal membrane of the proximal tubule, and their reabsorption within this nephron segment is of profound importance as some of these reabsorbed amino acids can act as gluconeogenic substrates.
In the intricate landscape of the renal system, the glomerulus assumes the role of a selective filter, allowing free amino acids to pass through, while the proximal tubule primarily shoulders the responsibility of reabsorbing these amino acids. The reabsorption process itself is a multifaceted interplay involving mechanisms such as diffusion, facilitated diffusion, and sodium-dependent active transport. Furthermore, the basolateral amino acid transporters are instrumental in orchestrating the reabsorption process, each serving specific physiological functions that contribute to the overall efficiency of renal amino acid handling.
Alternatively, amino acids may also embark on an oxidative journey, subsequently entering the tricarboxylic acid (TCA) cycle at distinct junctures. A noteworthy source of energy resides in branched-chain amino acids (BCAA), comprising leucine, valine, and isoleucine.
The branch chain α-ketoacid dehydrogenase (BCKDH) complex plays a pivotal role in the metabolic pathway of BCAAs, instigating an oxidative decarboxylation reaction subsequent to the initial transamination of BCAA by branched-chain aminotransferases (BCAT), yielding branched chain α-ketoacids. Metabolites stemming from the catabolism of BCAAs traverse through the oxidative pathways of the TCA cycle, leading to the formation of succinyl-CoA or acetyl-CoA. Notably, the kidney stands as the sole tissue, apart from the heart and brown fat, where the expression and activity of both BCAT and BCKDH are distinctly observed, underscoring the kidney’s distinctive role in the robust oxidative flux of BCAAs.
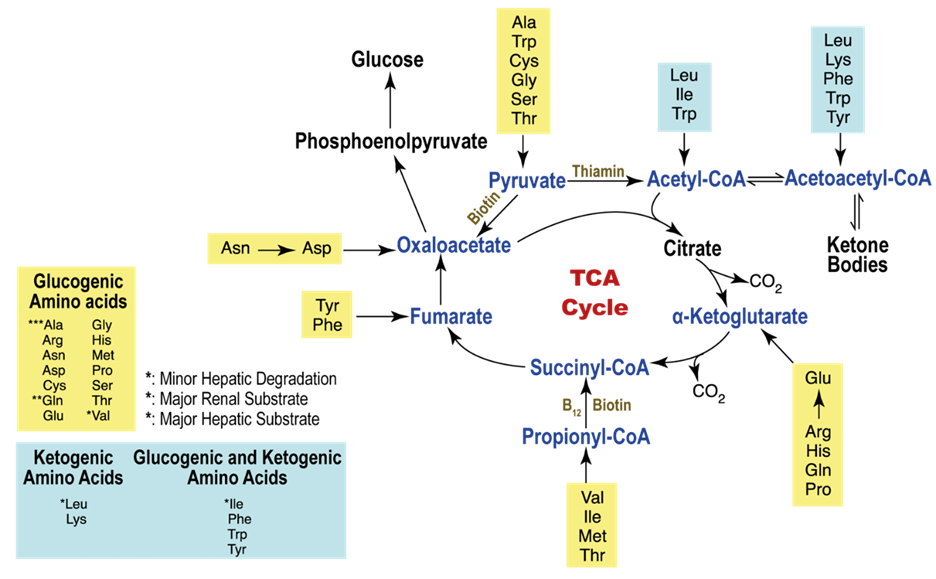
Hormonal Regulation of Energy Metabolism in Renal Cells
Insulin: Insulin, an endocrine hormone synthesized by the pancreas, holds a pivotal role in the meticulous control of glucose metabolism. Its influence extends to the kidneys, where it stimulates the uptake and utilization of glucose, thus aiding in the maintenance of optimal blood glucose levels.
Cortisol: Cortisol, a steroid hormone produced by the adrenal glands, is instrumental in the intricate orchestration of metabolic processes and energy equilibrium. Within the renal framework, cortisol fosters gluconeogenesis—a process by which new glucose is generated—and augments the catabolism of proteins and fats, channeling these molecules toward energy production.
Epinephrine and Norepinephrine: Epinephrine and norepinephrine, both emanating from the adrenal glands and intimately associated with the “fight or flight” response, impact renal function. These hormones stimulate the release of glucose by the kidneys and augment the degradation of glycogen, the body’s stored glucose reserves, to meet the immediate energy requirements imposed during stress or demanding situations.
This article has been prepared from the presentation of our student Deniz Usta.
References
Bhargava P, Schnellmann RG (2017). Mitochondrial energetics in the kidney. Nat Rev Nephrol., 13(10):629-646. doi: 10.1038/nrneph.2017.107.
Gewin LS (2021). Sugar or Fat? Renal Tubular Metabolism Reviewed in Health and Disease. Nutrients., 13(5):1580. doi: 10.3390/nu13051580.
National Institute of Diabetes and Digestive and Kidney Disease (NIDDK) (2023). Your Kidneys & How They Work.
Reece WO, Rowe EW (2017). Functional Anatomy and Physiology of Domestic Animals. 5th edition. Wiley-Blackwell
Sahay M, Kalra S, Bandgar T (2012). Renal endocrinology: The new frontier. Indian J Endocrinol Metab., 16(2):154-5. doi: 10.4103/2230-8210.93729.
Singh S, Sharma R, Kumari M, Tiwari S (2019). Insulin receptors in the kidneys in health and disease. World J Nephrol., 8(1):11-22. doi: 10.5527/wjn.v8.i1.11.
Published on: 26 October 2023Edited on: 26 October 2023